Trafficking of glucose, lactate, and amyloid-beta from the inferior colliculus through perivascular routes
- PMID: 19794399
- PMCID: PMC2801760
- DOI: 10.1038/jcbfm.2009.206
Trafficking of glucose, lactate, and amyloid-beta from the inferior colliculus through perivascular routes
Abstract
Metabolic brain imaging is widely used to evaluate brain function and disease, and quantitative assays require local retention of compounds used to register changes in cellular activity. As labeled metabolites of [1- and 6-(14)C]glucose are rapidly released in large quantities during brain activation, this study evaluated release of metabolites and proteins through perivascular fluid flow, a pathway that carries solutes from brain to peripheral lymphatic drainage sites. Assays with [3,4-(14)C]glucose ruled out local oxidation of glucose-derived lactate as a major contributor of label loss. Brief infusion of [1-(14)C]glucose and D-[(14)C]lactate into the inferior colliculus of conscious rats during acoustic stimulation labeled the meninges, consistent with perivascular clearance of [(14)C]metabolites from interstitial fluid. Microinfusion of Evans blue albumin and amyloid-beta(1-40) (Abeta) caused perivascular labeling in the inferior colliculus, labeled the surrounding meninges, and Abeta-labeled-specific blood vessels in the caudate and olfactory bulb and was deposited in cervical lymph nodes. Efflux of extracellular glucose, lactate, and Abeta into perivascular fluid pathways is a normal route for clearance of material from the inferior colliculus that contributes to underestimates of brain energetics. Convergence of 'watershed' drainage to common pathways may facilitate perivascular amyloid plaque formation and pathway obstruction in Alzheimer's disease.
Figures
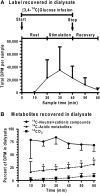
Similar articles
-
Astrocytes are poised for lactate trafficking and release from activated brain and for supply of glucose to neurons.J Neurochem. 2009 Oct;111(2):522-36. doi: 10.1111/j.1471-4159.2009.06333.x. Epub 2009 Aug 13. J Neurochem. 2009. PMID: 19682206 Free PMC article.
-
Cerebral amyloid angiopathy: amyloid beta accumulates in putative interstitial fluid drainage pathways in Alzheimer's disease.Am J Pathol. 1998 Sep;153(3):725-33. doi: 10.1016/s0002-9440(10)65616-7. Am J Pathol. 1998. PMID: 9736023 Free PMC article.
-
Astrocytic connexin distributions and rapid, extensive dye transfer via gap junctions in the inferior colliculus: implications for [(14)C]glucose metabolite trafficking.J Neurosci Res. 2007 Nov 15;85(15):3267-83. doi: 10.1002/jnr.21376. J Neurosci Res. 2007. PMID: 17600824 Free PMC article.
-
Cerebral amyloid angiopathy: accumulation of A beta in interstitial fluid drainage pathways in Alzheimer's disease.Ann N Y Acad Sci. 2000 Apr;903:110-7. doi: 10.1111/j.1749-6632.2000.tb06356.x. Ann N Y Acad Sci. 2000. PMID: 10818495 Review.
-
Perivascular drainage of amyloid-beta peptides from the brain and its failure in cerebral amyloid angiopathy and Alzheimer's disease.Brain Pathol. 2008 Apr;18(2):253-66. doi: 10.1111/j.1750-3639.2008.00133.x. Brain Pathol. 2008. PMID: 18363936 Review.
Cited by 33 articles
-
Extracranial Abscopal Responses after Radiation Therapy for Intracranial Metastases: A Review of the Clinical Literature and Commentary on Mechanism.Cureus. 2019 Mar 8;11(3):e4207. doi: 10.7759/cureus.4207. Cureus. 2019. PMID: 31114726 Free PMC article. Review.
-
Elimination of substances from the brain parenchyma: efflux via perivascular pathways and via the blood-brain barrier.Fluids Barriers CNS. 2018 Oct 19;15(1):30. doi: 10.1186/s12987-018-0113-6. Fluids Barriers CNS. 2018. PMID: 30340614 Free PMC article. Review.
-
Trajectories of Brain Lactate and Re-visited Oxygen-Glucose Index Calculations Do Not Support Elevated Non-oxidative Metabolism of Glucose Across Childhood.Front Neurosci. 2018 Sep 11;12:631. doi: 10.3389/fnins.2018.00631. eCollection 2018. Front Neurosci. 2018. PMID: 30254563 Free PMC article.
-
A systematic meta-analysis of oxygen-to-glucose and oxygen-to-carbohydrate ratios in the resting human brain.PLoS One. 2018 Sep 24;13(9):e0204242. doi: 10.1371/journal.pone.0204242. eCollection 2018. PLoS One. 2018. PMID: 30248124 Free PMC article.
-
The Glymphatic System in Central Nervous System Health and Disease: Past, Present, and Future.Annu Rev Pathol. 2018 Jan 24;13:379-394. doi: 10.1146/annurev-pathol-051217-111018. Annu Rev Pathol. 2018. PMID: 29195051 Free PMC article. Review.
Publication types
MeSH terms
Substances
Grant support
LinkOut - more resources
-
Full Text Sources
-
Other Literature Sources