- Journal List
- HHS Author Manuscripts
- PMC2588647

Novel biomarkers for risk of prostate cancer
Li Yang
1Eppley Institute for Research in Cancer and Allied Diseases, University of Nebraska Medical Center, Omaha, Nebraska
Nilesh W. Gaikwad
1Eppley Institute for Research in Cancer and Allied Diseases, University of Nebraska Medical Center, Omaha, Nebraska
Jane Meza
2Department of Biostatistics, University of Nebraska Medical Center, Omaha, Nebraska
Ercole L. Cavalieri
1Eppley Institute for Research in Cancer and Allied Diseases, University of Nebraska Medical Center, Omaha, Nebraska
Paola Muti
3Department of Cancer Epidemiology, Italian National Cancer Institute, Rome, Italy
Bruce Trock
4Brady Urological Institute, The Johns Hopkins School of Medicine, Baltimore, Maryland
Eleanor G. Rogan
1Eppley Institute for Research in Cancer and Allied Diseases, University of Nebraska Medical Center, Omaha, Nebraska
5Department of Environmental, Agricultural and Occupational Health, University of Nebraska Medical Center, Omaha, Nebraska
Abstract
Background
Although the estrogens estrone and estradiol are recognized to play very important roles in the risk of developing prostate cancer (Pca), the molecular mechanism by which estrogens initiate and/or promote Pca is still unknown. Substantial evidence supports that specific metabolites of estrogens, catechol estrogen quinones, can react with DNA to form depurinating estrogen-DNA adducts. Apurinic sites derived from depurination of these adducts can induce mutations leading to cancer. Once released from DNA, depurinating estrogen-DNA adducts are shed from cells into the bloodstream and excreted in urine. By analyzing profiles of estrogen metabolites, conjugates, and depurinating DNA adducts in urine from men with and without prostate cancer, potential biomarkers of Pca can be detected. The goal of this case-control study was to detect and identify potential biomarkers of Pca.
Methods
Urine samples from fourteen cases, men diagnosed with Pca, and 125 controls, men who had not been diagnosed with Pca, were partially purified by solid phase extraction and analyzed by ultraperformance liquid chromatography/tandem mass spectrometry. The urinary levels of androgens, estrogens, estrogen metabolites, conjugates and depurinating DNA adducts were measured.
Results
The ratio of depurinating estrogen-DNA adducts to the sum of the corresponding estrogen metabolites and conjugates was significantly higher in cases (median: 57.34) compared to controls (median: 23.39) (p<0.001).
Conclusions
This study suggests that depurinating estrogen-DNA adducts could serve as potential biomarkers to predict risk of Pca. They also could be useful tools for early clinical diagnosis and development of suitable strategies to prevent Pca.
Introduction
Prostate cancer (Pca) has been a significant public health concern for a long time due to its high frequency of diagnosis, and it is the second leading cause of cancer deaths in men in the United States. Approximately 186,320 new cases and 28,660 deaths from Pca are estimated to occur in the U.S. in 2008 (http://seer.cancer.gov). The etiology of this disease is not yet understood; however, several risk factors, such as age, endocrine status, genetic susceptibility, and ethnicity have been identified [1-6].
Substantial laboratory data suggest that androgens play an important role in susceptibility to Pca; however, most epidemiologic data in humans are inconclusive [7-12]. It has been suggested that aromatization of androgens to estrogens may play a role in prostate carcinogenesis or tumor progression [13-15]. The potential role of estrogens in the etiology of Pca has been recognized only recently, although estrogens play a physiologic role during prostate development, growth and differentiation [16,17]. In the Noble rat model, Leav and associates demonstrated that long-term administration of testosterone plus estradiol (E2) in adulthood leads to a high incidence of adenocarcinomas in the dorsolateral prostate [18]. In a neonatally estrogenized rodent model, prostatic hyperplasia and dysplasia are prominent with aging, and prostatic intraepithelial neoplasia is observed when these rats are given exogenous testosterone [19-23]. Protracted androgen-supported, estrogen-enhanced stimulation of cell proliferation may be required for dysplastic lesions to develop in the rat prostate [15]. Epidemiological data show that men with high levels of estrogens have a greater risk of Pca [13,24,25]. Experimental results and some epidemiological data support a role of estrogens in the etiology of this disease. The specific role of estrogens has not been established, although data suggest that estrogens might initiate Pca [26].
Exposure to high levels of estrogens is a well established risk factor for breast cancer [27]. Mounting in vitro and in vivo evidence supports the hypothesis that specific estrogen metabolites, namely catechol estrogen quinones, can react with DNA to form depurinating estrogen-DNA adducts. Apurinic sites that are formed by depurination of these adducts can induce mutations leading to cancer [28]. Prostate and breast cancer share many similarities, such as risk factors, biomolecular determinants, geographical distribution and natural history of disease [17]. This indicates that estrogens might play a role in the etiology of prostate and breast cancer with a similar mechanism. Recently, it was shown in a case-control study that elevated depurinating estrogen-DNA adducts in urine are associated with a high risk of breast cancer [29]. The 4-hydroxyestrone-1-N3Ade (4-OHE1-1-N3Ade) adduct was also detected at higher levels in urine samples from Pca cases and benign urological conditions compared to controls [26]. Enzymes involved in the estrogen metabolic pathway are expressed in the prostate. Cytochrome P450 (CYP)1A1 was detected in the majority of twelve prostate tissue sets and higher CYP1B1 expression in the peripheral zone than in the transition zone in the prostate was also observed, which suggests that CYP1B1 may be associated with risk of Pca [30]. In addition, the potential overexpression of CYP1B1 could be the source of unbalanced estrogen metabolism in the prostate [31]. The quinone reductase NQO1 gene, which is also involved in estrogen metabolism, may be associated with increased risk of Pca [32].
E1 and E2 are metabolized mainly to 2- and 4-catechol estrogens (CE) by CYP1A1 and CYP1B1 in extrahepatic tissues [33]. We hypothesize that estrogens initiate Pca mainly by formation of 4-CE, followed by their oxidation to catechol estrogen-3,4-quinones (CE-3,4-Q), which can react with DNA to form depurinating adducts (Fig. 1). To avoid cancer initiation, CE can be detoxified by catechol-O-methyltransferase (COMT), and CE-3,4-Q by conjugation with glutathione (GSH) or by reduction to CE, catalyzed by quinone reductase (NQO1 and/or NQO2 [34,35]) and/or cytochrome P450 reductase. If the metabolism of estrogens is not kept in balance, the risk of developing Pca will increase. Thus, estrogen metabolites, GSH conjugates and/or depurinating DNA adducts in urine samples from Pca cases and controls are hypothesized to reflect the estrogen metabolism in the body, and their levels are expected to be different. Thus, they could be used to determine risk of Pca.
The purpose of this case-control study was to evaluate the possible role of estrogen metabolism in the pathogenesis of Pca by investigating the urinary androgens, estrogens, estrogen metabolites, conjugates and depurinating DNA adducts in men with and without Pca. The profile of estrogen metabolites, conjugates and depurinating DNA adducts in urine from cases and controls reflects differences in estrogen metabolism. Thus, biomarkers of Pca may be identified.
Materials and Methods
Study subjects
This Pca case-control study included men from 45 to 83 years of age that were residents of the U.S. The urine samples were collected from two sources: the Johns Hopkins Medical Institute (JHMI) and the School of Medicine and Biomedical Science at the University of Buffalo [Western New York Health Cohort Study (WNYHCS)].
Eligibility criteria for all the subjects were age greater than 45 and negative for family history of Pca. Fourteen cases more than 50 years of age from JHMI were enrolled. The eligibility criteria for cases were incidental diagnosis of Pca and histopathological and/or cytological confirmation. Cases were recruited from January 2006 to December 2006.
The control group included 125 men who were free of Pca at the time the urine sample was collected. In the control group, seven were enrolled from JHMI and 118 from the WNYHCS. The control subjects were eligible because they were free of Pca. The pertinent medical records by the related urologists were requested and obtained. One hundred and eighteen WNYHCS controls were recruited from 1994-2001, and seven controls from JHMI were recruited from January 2006 to December 2006. At the time of recruitment, data on demographics and life style, such as smoking and alcohol habits, were collected.
Sample Collection
A morning spot urine sample was collected from each participant and 1 mg/ml ascorbic acid was added to prevent oxidation of the catechol moieties in the various estrogen compounds. The urine samples were aliquoted, frozen and transferred to the Eppley Institute, University of Nebraska Medical Center (UNMC), on dry ice and stored at -80 °C until analysis.
Laboratory Analysis
To minimize bias, the collection time (morning), storage (-80 °C) and transport (using dry ice) of all urine samples were standardized. Laboratory personnel were blinded to the case-control status. The samples were randomly chosen as the same batch, handled identically and run on the same day under the same instrument conditions. All of the study samples were analyzed in duplicate.
Two-milliliter aliquots of urine underwent partial purification by solid phase extraction (SPE) with a phenyl cartridge (Varian, Palo Alto, CA). The cartridges were pre-conditioned with methanol and the loading buffer, 10 mM ammonium formate, pH 7. Before being loaded onto the pre-conditioned cartridges, the urine samples were adjusted to pH 7. After washing with loading buffer, the target compounds were eluted from the cartridge by using an elution buffer, methanol/10 mM ammonium formate, pH 7 (90:10) with 1% acetic acid. The collected eluates were concentrated with a SpeedVac and lypholizer. The eluates were redissolved in methanol/distilled water (50:50) and subjected to ultraperformance liquid chromatography/tandem mass spectrometry (UPLC/MS-MS) analysis. The analytes were identified by their retention time and by tandem mass spectrometry. Treatment of urine with glucuronidase/sulfatase led to significant increases (10-20 fold) in the levels of E1 and E2, while the levels of estrogen metabolites, conjugates and adducts changed marginally and in many cases decreased because of the lengthy incubation. To avoid artifacts and errors that are introduced by maintaining the urine samples at 37 °C for 8 h, we carried out all the analyses without glucuronidase/sulfatase treatment.
UPLC/MS-MS analyses were carried out with a Waters Acquity UPLC system connected with a high performance Quattro Micro triple quadrupole mass spectrometer. Analytical separations on the UPLC system were conducted using an Acquity UPLC BEH C18 1.7 μm column (1 × 100 mm) at a flow rate of 0.15 ml/min. The gradient started with 80% A (0.1% formic acid in H2O) and 20% B (0.1% formic acid in CH3CN), changed to 79% A over 4 min, followed by a 6-min linear gradient to 45% A, resulting in a total separation time of 10 min. The elutions from the UPLC column were introduced to the Quattro Micro mass spectrometer. The ionization method used for MS analysis was electrospray ionization (ESI) in both positive ion (PI) and negative ion (NI) mode. ESI-MS capillary voltage was 3.0 kV, extractor cone voltage was 2 V, and detector voltage was 650 V. Desolvation gas flow was maintained at 600 l/h. Cone gas flow was set at 60 l/h. Desolvation temperature and source temperature were set to 200 and 100 °C, respectively. MS-MS was performed in the multiple reaction monitoring (MRM) mode to produce structural information about the analytes by fragmenting the parent ions inside the mass spectrometer and identifying the resulting daughter/fragment ions. Resulting data were processed by using QuanLynx software (Waters) to quantify the estrogen metabolites. All solvents used were of HPLC grade.
The 40 analytes included the androgens androstenedione and testosterone; the estrogens E1-sulfate, E1 and E2; the catechol estrogens 2-OHE1(E2) and 4-OHE1(E2); the 16α-OHE1(E2); the methylated 2- and 4-catechol estrogens 2-OCH3E1(E2) and 4-OCH3E1(E2); the 2- and 4-catechol estrogens conjugated with GSH, cysteine (Cys) or N-acetylcysteine (NAcCys): 2-OHE2-1-SG, 2-OHE2-4-SG, 2-OHE1-1-SG, 2-OHE1-4-SG, 2-OHE2-(1+4)-Cys, 2-OHE1-1-Cys, 2-OHE1-4-Cys, 2-OHE2-1-NAcCys, 2-OHE2-4-NAcCys, 2-OHE1-1-NAcCys, 2-OHE1-4-NAcCys, 4-OHE2-2-SG, 4-OHE1-2-SG, 4-OHE2-2-Cys, 4-OHE1-2-Cys, 4-OHE2-2-NAcCys and 4-OHE1-2-NAcCys; and the depurinating DNA adducts of 4-OHE1(E2) and 2-OHE1(E2): 4-OHE2-1-N7Gua, 4-OHE1-1-N7Gua, 4-OHE2-1-N3Ade, 4-OHE1-1-N3Ade, 2-OHE2-6-N3Ade and 2-OHE1-6-N3Ade.
The ratio of adducts to their respective metabolites and conjugates was calculated according to following formula:
We previously provided a detailed description of this method and its reliability assessment and limit of detection [29].
Statistical Analysis
Data represent the mean of two independent experiments for each subject. The target compounds in the urine were normalized by total urinary creatinine. The ratio of depurinating estrogen-DNA adducts to the sum of their corresponding metabolites and conjugates was analyzed as a continuous variable. We examined distributions for all variables of interest. Non-parametric tests were used when departures from normality were observed.
Androgens, estrogen-related compounds, ratio of adducts to metabolites and conjugates, and age were compared for Pca cases vs. controls using the Mann-Whitney U test.
To compute an odds ratio of Pca in relation to the ratio of depurinating estrogen-DNA adducts to the sum of its corresponding metabolites and conjugates with Fisher’s exact test, we used a cut-off value of 50 for the ratio.
For all analyses, a two-sided p-value < 0.05 was interpreted as a statistically significant difference. All statistical analyses were conducted using SPSS (SPSS Inc. Chicago, IL) and Prizm software.
Results and Discussion
Descriptive analysis of study subjects
In this study men with and without Pca were compared, despite having a small number of cases (n=14) and a larger number of controls (n=125). The mean age of cases was 63.6 years, median was 60 years, and the range was from 50-83 years. The mean age of controls was 64.8 years, median was 67 years, and the range was from 45-78 years. All fourteen cases were Caucasian, and 123 controls were Caucasian, with two being black. Since the eligibility for both cases and controls excluded males with a family history of Pca, cases and controls were matched on age.
Urine data analysis
The values obtained for the various androgens and estrogen-related compounds in cases and controls were analyzed in two different ways. First, median values were compared for all the compounds in urine samples for the two groups of men (Table 1). Then, we compared the ratio of depurinating estrogen-DNA adducts to the sum of their corresponding estrogen metabolites and conjugates in urine samples from Pca cases and controls because the ratio reflects the degree of imbalance in estrogen metabolism that can lead to cancer initiation.
Table 1
No. | Compound | Prostate Cancer (n=14) | Control (n=125) | p-value** | ||
---|---|---|---|---|---|---|
pmol/ml | ||||||
Median | Min-Max | Median | Min-Max | |||
1 | Androstenedione | 9.0 | 3.3-21.8 | 8.3 | 1.9-24.7 | |
2 | Testosterone | 2.5 | 0.9-5.8 | 2.0 | 0.1-9.2 | |
3 | E1-sulfate | 1.4 | 0.2-14.3 | 1.4 | 0.0-17.8 | |
4 | E2 | 12.8 | 4.9-36.2 | 11.2 | 0.0-48.3 | |
5 | E1 | |||||
6 | 2-OHE2 | 8.1 | 3.2-86.1 | 9.4 | 1.0-116 | |
7 | 2-OHE1 | |||||
8 | 4-OHE2 | 6.1 | 0.4-14.3 | 7.1 | 0.0-120. | |
9 | 4-OHE1 | |||||
10 | 16α-OHE2 | 106 | 38-549 | 114 | 7-954 | |
11 | 16α-OHE1 | |||||
12 | 2-OCH3E2 | 35.0 | 4.8-71.7 | 23.3 | 0.6-290 | |
13 | 2-OCH3E1 | |||||
14 | 4-OCH3E2 | 13.7 | 4.1-27.8 | 12.3 | 1.1-511 | |
15 | 4-OCH3E1 | |||||
16 | 2-OH-OCH3E2 | |||||
17 | 2-OH-OCH3E1 | |||||
18 | 2-OHE2-1-SG | 7.2 | 1.7-12.7 | 5.6 | 1.0-27.4 | |
19 | 2-OHE2-4-SG | |||||
20 | 2-OHE1-1-SG | |||||
21 | 2-OHE1-4-SG | |||||
22 | 2-OHE2-1+4-Cys | |||||
23 | 2-OHE1-1-Cys | |||||
24 | 2-OHE1-4-Cys | |||||
25 | 2-OHE2-1-NAcCys | |||||
26 | 2-OHE2-4-NAcCys | |||||
27 | 2-OHE1-1-NAcCys | |||||
28 | 2-OHE1-4-NAcCys | |||||
29 | 4-OHE2-2-SG | 4-OHE2-2-Cys | 3.2 | 0.8-6.5 | 3.6 | 0.4-18.7 |
30 | 4-OHE1-2-SG | |||||
31 | 4-OHE2-2-Cys | |||||
32 | 4-OHE1-2-Cys | |||||
33 | 4-OHE2-2-NAcCys | |||||
34 | 4-OHE1-2-NAcCys | |||||
35 | 4-OHE2-1-N7Gua | 0.7 | 0.4-1.6 | 0.3 | 0.0-2.9 | 0.0004 |
36 | 4-OHE1-1-N7Gua | |||||
37 | 4-OHE2-1-N3Ade | 0.4 | 0.1-3.1 | 0.2 | 0.0-3.0 | 0.056 |
38 | 4-OHE1-1-N3Ade | |||||
39 | 2-OHE2-6-N3Ade | 0.2 | 0.0-1.8 | 0.0 | 0.0-1.8 | 0.0014 |
40 | 2-OHE1-6-N3Ade |
Comparison of median values of the urinary androgens and estrogen-related compounds from Pca cases and controls
There was no evidence of a difference in the median levels of andostenedione, testosterone or E1-sulfate in the urine from cases and controls (Table 1). The median levels of E1 and E2 in cases and controls were not compared because this analytical method, which does not include treatment with glucuronidase/sulfatase, detects only a small portion of these estrogens. The median levels of 16α-OHE1(E2), 2-OHE1(E2) and 4-OHE1(E2) from the two groups were not significantly different (Table 1).
There was no significant difference in the median levels of 2-OCH3E1(E2), 4-OCH3E1(E2) or thiol conjugates between the two groups.
The median levels of 4-OHE1(E2)-1-N7Gua, 4-OHE1(E2)-1-N3Ade and 2-OHE1(E2)-6-N3Ade, which reflect the oxidative pathway of estrogen metabolism, are higher in the Pca group than those in the control group (p=0.0004, p=0.056, p=0.0014, respectively). These medians and their distribution are shown graphically in Figure 2, and suggest that the oxidative pathway that leads to DNA adduct formation is more active in the Pca group than in the control group.
Comparison of median values of the ratios of depurinating DNA adducts to the sum of their corresponding metabolites and conjugates in urine from cases and controls
The ratio of depurinating estrogen-DNA adducts to the sum of their corresponding estrogen metabolites and conjugates reflects estrogen metabolism in the body. Estrogens can be oxidized to their catechol estrogen quinones, which can react with DNA to form 4-OHE1(E2)-1-N3Ade, 4-OHE1(E2)-1-N7Gua and 2-OHE1(E2)-6-N3Ade adducts, although the oxidative pathway of estrogen metabolism can be blocked by reduction, methoxylation and/or conjugation of the metabolites. When the balance between activating and deactivating pathways of estrogen metabolism is disrupted, the oxidation pathway can prevail and the level of depurinating estrogen-DNA adducts will be high, while the protective pathway is diminished and relevant protective compounds, such as 4-OCH3E1(E2) and 4-OHE1(E2)-thiol conjugates, will be low. Thus, the ratio can increase when estrogen metabolism is unbalanced.
We analyzed the ratio of depurinating N3Ade and N7Gua adducts to the sum of their corresponding estrogen metabolites and conjugates in urine samples as a continuous variable. Analysis by the Mann-Whitney U test revealed a significant difference between the two groups (p<0.001) (Fig. 3). The above mentioned ratio is significantly higher in the Pca cases (57.34) than in the control group (23.39), which is consistent with the hypothesis that these adducts are a causative factor in the etiology of Pca.
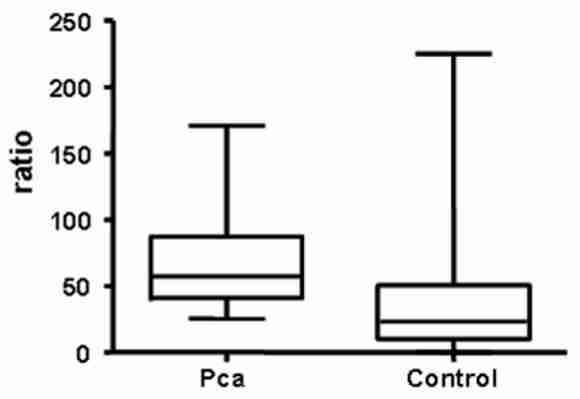
Ratio of depurinating DNA adducts to the sum of the corresponding estrogen metabolites and conjugates in Pca cases and controls. The Pca group contained 14 subjects, and the control group, 125 subjects. Median values and ranges are presented.
In the sum of the ratios of depurinating adducts to estrogen metabolites and conjugates, the predominant contribution comes from the N3Ade and N7Gua adducts of 4-OHE1(E2), whereas the adducts of 2-OHE1(E2) play a very minor role. These data are consistent with earlier publications in which the 4-OHE1(E2) metabolism pathway plays a major role in the initiation of cancer [26,28,29].
Odds ratio analysis of Pca risk using the ratio of depurinating estrogen-DNA adducts to their corresponding metabolites and conjugates as a biomarker
With a cut-off value of 50 for the ratio of depurinating estrogen-DNA adducts to their corresponding metabolites and conjugates, we used Fisher’s exact test to analyze the odds ratio of Pca risk. The resulting odds ratio is 7.58 (95% confidence interval: 2.22 to 25.91), p <0.001). For subjects with a ratio of depurinating estrogen-DNA adducts to their corresponding metabolites and conjugates higher than 50, their risk of Pca is estimated to be nearly 8 times that of subjects with a ratio lower than 50. With a cut-off value of 50, the sensitivity is 0.71 with 95% CI of 0.42 to 0.92, and the specificity is 0.75 with 95% CI of 0.67 to 0.82. Using a cut-off value of 25 or 75, the odds ratio is unsatisfied, or the specificity or sensitivity is unsatisfied (with a cut-off value of 25 for the ratio, specificity is 0.46; with cut-off value of 75, sensitivity is 0.29).
Conclusions
In this study, urine samples from men with Pca had relatively high levels of depurinating estrogen-DNA adducts compared to urine samples from healthy control men. These results suggest that the depurinating estrogen-DNA adducts could serve as potential biomarkers to predict risk of Pca. The strength of this study is that it is the first to investigate the whole profile of estrogen metabolism in Pca cases and healthy controls, as well as the association of unbalanced estrogen metabolism with risk of Pca. This study suggests that the depurinating estrogen-DNA adducts could be useful tools for early clinical diagnosis and development of suitable strategies to prevent Pca. Since it had a small sample size, future large-scale studies are needed to confirm these results.
Acknowledgments
Grant sponsor and grant number: Supported by Prevention LLC and by Department of Defense grant DAMD17-02-1-0660. Core support at the Eppley Institute was provided by grant P30 CA36727 from the National Cancer Institute.